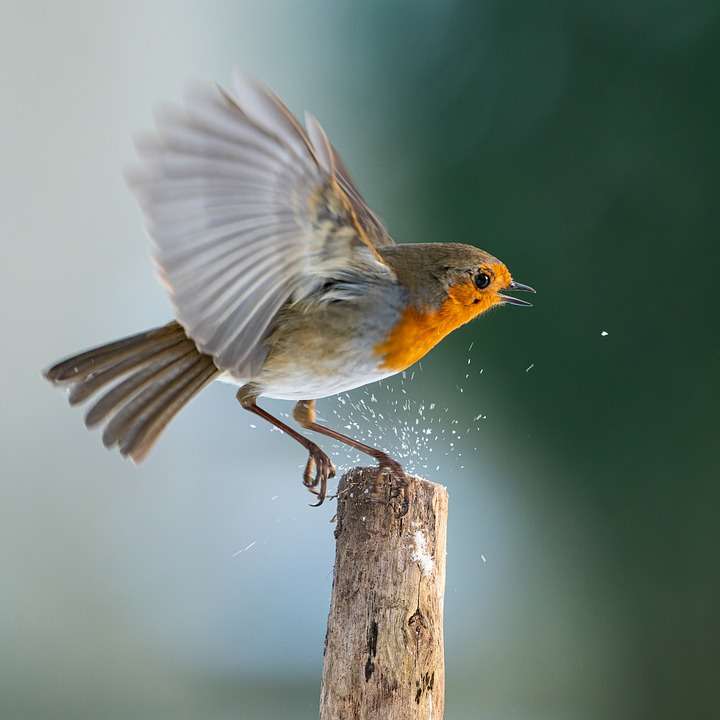
The geomagnetic field as a source of directional information
The Earth, due to its constituent characteristics, is comparable to a huge magnet. We live like this, like it or not, immersed in the lines of force of its magnetic field. These envelop our planet starting from a point close to the geographical south pole, to return close to the north one, thus constituting the magnetic terrestrial poles. In their path, the field lines assume continuously variable angles of inclination with respect to the horizontal, running parallel to the Earth’s surface only near the magnetic equator (Fig. 1).
Furthermore, the field intensity varies significantly: it decreases from 60,000 to 30,000 nT (Tesla is the magnetic unit of measurement) going from the magnetic poles to the equator. Furthermore, the trend of the lines of force is complicated by local deviations depending on the composition of the materials of the earth’s crust. In fact, ferrous rocks distort them considerably and, just to give an example, those who go to sea know well that our compasses do not tell the truth in the vicinity of the island of Elba, due to the ferrous deposits that constitute the substrate.
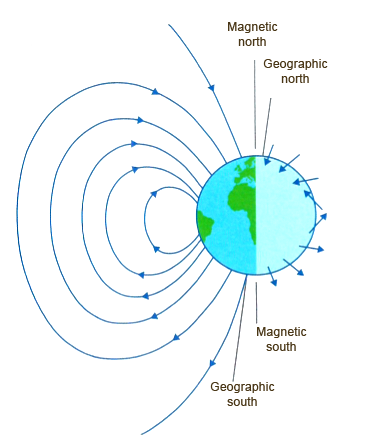
Our compasses simply align their magnetized needles with the field lines, thus invariably indicating magnetic north. Any animal that was sensitive to the geomagnetic field would then be able to obtain directional information from it: therefore having a magnetic compass.
That animals are sensitive to magnetic orienting stimuli is largely beyond doubt: just observe in Figure 2 what happens to the orientation behaviour of passerine birds if we rotate the position of the magnetic north pole perceived by them: in spring a migrating robin orients itself northward, i.e. its correct flight direction (2b). If using Helmholtz spirals, placed around its cage, we rotate the magnetic north towards the ESE, its directional choices also deviate in this direction (2a). The same happens if the north is placed in the west (2c). Likewise, a passenger pigeon may have difficulty orienting itself toward home under cloudy skies from places with strong field line deviations, as I observed in launches from the volcanic area of Viterbo, in a similar way to what was done by colleagues at Cornell University (NY) from a place called, coincidentally, Iron Mine.
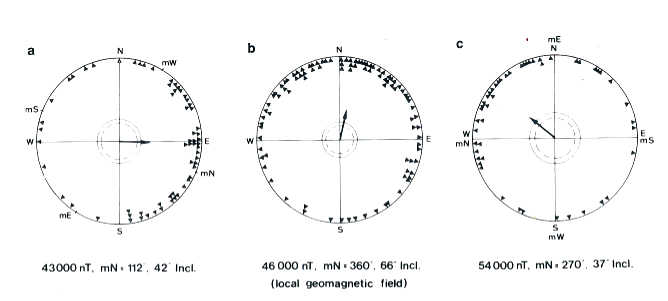
If the behavioral response of birds leaves no doubt about their magnetic sensitivity, there are however some doubts in this regard, mainly due to two reasons:
- no one has managed to condition birds to magnetic stimuli;
- we don’t know which receptor is responsible for magnetic sensitivity, and this is the case for any species.
From Pavlovian-type experiments, we know that a bird can be conditioned by sound, visual, or electrical stimuli to perform a certain movement or action, but none of them have ever responded to magnetic stimulation.
Similarly, the search for a “magnetic receptor” has long occupied physiologists and anatomists, so far without appreciable success. There are many theories but the experimental results are hardly encouraging and none are certain. Today the most promising hypothesis is based on a mechanism mediated by proteins (cryptochromes) present in the retina of birds and mammals. However, among the various subtypes of cryptochromes present in different parts of the retina, a sure candidate as a primary protein for magneto reception has not yet been identified. The nervous pathway that should carry retinal information to the decision-making centers of the brain is even more uncertain.
But then does a magnetic compass exist or not? And what are its functional characteristics? Even though the sun, moon and stars are my favorite sources of directional information, I certainly cannot deny the role of geomagnetism as a primary and innate compass element in animals, not needing to be learned and even functioning as a calibration system for the other compasses just mentioned.
The problem of how magnetic stimuli can be read and used for compass purposes was solved by Wolfang and Rosita Wiltschko of the University of Frankfurt in 1972 (The magnetic compass of European robin. Science 172: 62-64). Their experiments on the robin highlighted two fundamental differences compared to the compasses we use. The first fact is that the operating range of the compass is tuned to the intensity of the field at that point. A sudden change in intensity blocks the compass reading. A migrant must therefore adapt to the field intensities gradually encountered along the journey, a fact which is not immediate but takes time (2-3 days). Second, if our compasses are “oriented by polarity” by reading the direction of the field which is always north, those of birds are “oriented by inclination” by paying attention to the angle that the field lines make with the earth’s surface at that point.
The inclination parameter varies angularly between 90° at the magnetic equator and 0° at the poles, as the field lines are horizontal and respectively vertical at the two points. Furthermore, the vector representing it is directed upwards in the southern hemisphere and downwards in the northern hemisphere (compare with figures 1 and 3). According to this parameter, birds do not distinguish between north and south, but rather between “poleward” or “equatorward” magnetic.
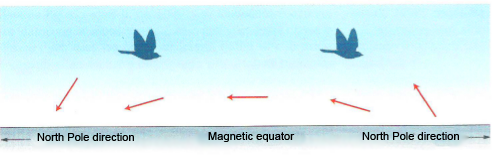
The inclination vector parameter is therefore variously oriented to the earth’s surface, i.e. it forms a certain angle with the gravity vector. We then see in Figure 4 how the Wiltschkos hypothesized the functioning of the magnetic compass in the robin: in the northern hemisphere, it recognizes as “northward” the direction in which the inclination and gravity vectors form the most acute angle α (4a). It thus recognizes the route to take in spring to reconquer the European nesting areas. If we experimentally invert the horizontal and vertical components of the inclination vector, there is no effect on the orientation of the robin as the smaller angle is in the usual direction (4b). If only the vertical component (4c), or the horizontal one (4d) is inverted, the situation of the southern hemisphere is reproduced, and the directional preferences of the robin are inverted, indicating the direction “towards the equator” that the robin travels in fall.
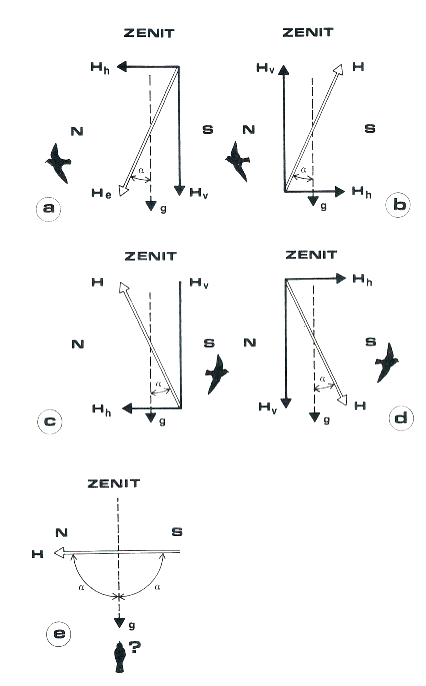
In equatorial conditions in which the inclination vector is parallel to the surface and therefore perpendicular to gravity, two 90° angles are formed, in which case the robin does not know how to take any direction (4e). The robin does not migrate beyond the equator, remaining to winter in the Mediterranean area, but for trans-equatorial migrants who should use the same type of compass, how can they manage the passage in peri-equatorial areas? The Wiltschkos themselves admit that from a compass point of view this “is still an open question”. For warblers, warblers, and pied flycatchers, the transition to a star compass (as they are nocturnal migrants) is perhaps the easiest hypothesis, even if not so in overcast skies…
The lack of alternative models of how a magnetic compass works has meant that the one proposed by the Wiltschkos has been generally accepted for birds, but as perhaps can be seen from these lines, there are still many questions to be answered.
Credits
Author: N. Emilio Baldaccini. Former Professor of Ethology and Conservation of Zoocenotic resources at University of Pisa. Author of over 300 scientific papers on national and international journals. He is active in the field of scientific education, and co-author of academic textbooks of Ethology, General and Systematic Zoology, Comparative Anatomy.
Translation by Maria Antonietta Sessa